Section 4 Tree recruitment
Sustainable natural regeneration of forests will help ensure resilience in a changing climate, and as such it is a primary focus of forest management prescriptions. There are innumerable factors affecting the process of regeneration and recruitment, including weather, climate, disturbances, soil conditions, and competition from other seedlings and other species. As climate change rapidly raises temperatures and may change patterns of precipitation [including snow12] in the Southwest, the conditions for successful regeneration and recruitment of some tree species may be at significant risk. Typically, the climatic conditions required for seedling germination and survival are wetter and cooler than is necessary for growth and survival of adult trees59. Seedlings are far more prone to drought-induced mortality than adult trees because of shallower root systems, increased competition, and fewer stored and available resources. Likewise, their small stature and thin bark make them far less resistant to fire, so that if climate change will continue to make wildfires more frequent, larger, and more severe, there is a real and inherent risk of losing seedlings at extremely high rates.
The forests of the Defiance Plateau and Chuska Mountains of the Navajo Nation are dominated by ponderosa pine. Ponderosa pine tends to regenerate in sporadic pulses that can be regionally widespread given optimal climate conditions60. With the variable precipitation regime of the Southwest, ponderosa pine appears to require a series of above-average wet, warm summers and mild winters that enable successful flowering, cone development, and seed germination61,62. Disturbances, particularly surface fires, are also important in preparing the soil seedbed by removing litter and duff and limiting competition from grasses and shrubs. Recurrent fires also thin clumps of developing seedlings. All of this makes for dynamic relationships among tree recruitment, fire, and climate.
The mix of conditions that are most conducive to tree regeneration (seed germination and growth) and recruitment (survival to adulthood) appear to be place and time dependent, with research pointing to safe spaces63 as well as safe time periods64,65. The relative safety in space and time allow for seedlings and saplings to develop traits that promote fire resistance, including height and thick bark. There is currently no consensus on what mix of climate and disturbance regimes are best for managing for recruitment, emphasizing the need for further place-based study of forest regeneration dynamics.
The Navajo demography project was designed to answer a key management concern raised by the Navajo Forestry Department: When will our next big tree cohort come? If only we could foresee the future this would be an easy answer, but of course that’s simply not an option. Instead, we can look to the past to learn of patterns and processes that established tree cohorts historically, and use those parameters to help in predicting how ponderosa pine forests can (or cannot) continue to be sustainable in a warmer, drier future.
The demography project includes three sites selected in partnership with the Navajo Forestry Department to represent the elevation range, forest composition, and forest structure of once-common old-growth forests on the Defiance Plateau and Chuska Mountains (Figure 4.1). Each site is approximately 100 hectares (250 acres) in size and includes 7 randomly distributed plots. The plots consist of the 25-30 nearest live or dead trees meeting a minimum size of 10 cm (4 inches) diameter at breast (DBH; 4.5 feet above ground). At the Falling Irons (FFe) and Tohatchi Lookout (TLK) sites, we found a high-density of young trees (< 125 years old, known as black jacks), and divided the plots into two subplots: an inner plot including the nearest 5 trees per species estimated to be older than 1880, and an outer plot including the nearest 20 trees older than 1880. The cut-off for tree age at 1880 was used because it represents the end of frequent fire activity across the study area (see Historical fire regimes and 44). Trees younger than 1880 have several distinguishing features: (1) They are usually among the smallest trees, (2) they have few if any bark characteristics that appear with age (for example, ponderosa pine develop orange to yellow bark coloration at ~125 years old on this landscape), and (3) they often have low hanging branches that have not been pruned off by exposure to heat from surface fires (in fact, most younger trees have not been exposed to fire). For each tree on the plots, we recorded species, status (live, stump, snag, or log), geographic position (with a GPS as well as the tree azimuth and distance to plot center), and DBH. For live trees, we also recorded total height, crown base height, live crown ratio, and social position. We collected tree-ring specimens from live trees at ~10 cm above ground with an increment borer, and on dead trees at the approximate ground level with a chainsaw. Every effort was made to obtain the pith (inside most) ring, but on many live trees we tried until we were confident the core was within 10 rings of center. In addition to the plot samples, we sampled fire-scarred trees on, around, and between plots by searching for trees with well-preserved fire scars.
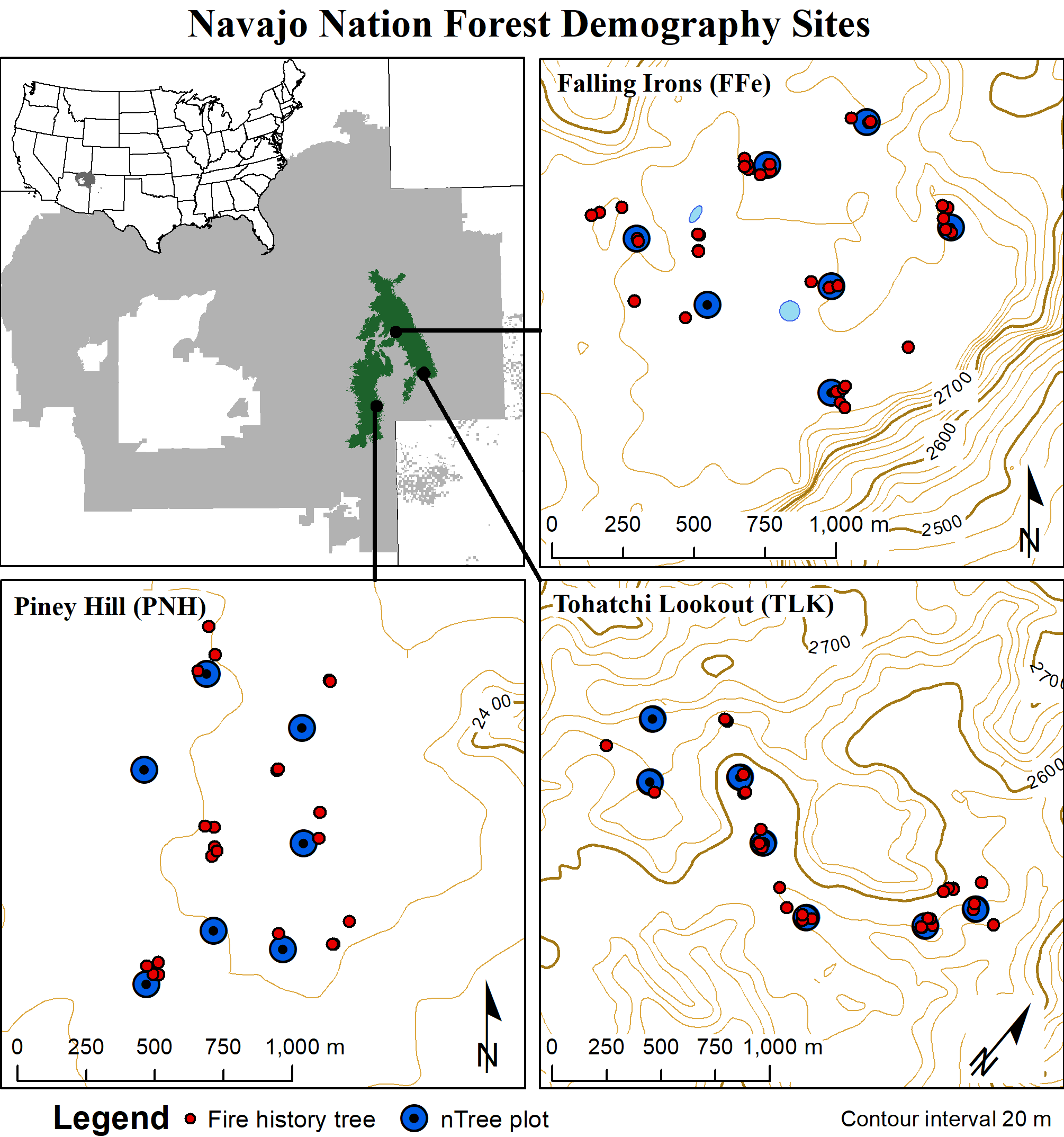
Figure 4.1: Site maps for the Navajo Demography Project. Inset maps at top-left show the location of the Navajo Nation and the study area of the Defiance Plateau-Chuska Mountains.
We followed standard methods of dendrochronology to sand and precisely date the tree-ring samples under a binocular microscope66. When the pith ring was not on the core or cross-section, we estimated the number of missing rings with a concentric circle method67. We then applied corrections to the dated and estimated pith dates to account for growth to sampling height. We calculated an average growth rate of two years per 10 cm height, based on estimates obtained from a selection of fire-scarred trees with at least two samples. This growth rate is in accord with comparable studies from nearby locations68,69. All fire scars were dated to the year and often the season in which they occurred41.
Our total tree-ring collection from the three demography sites included 585 trees (605 samples) on plots, with an additional 81 trees sampled outside of plots for fire history reconstruction, and 29 trees sampled for chronology building purposes. Our analyses include 551 crossdated trees from the plots (94%) and 69 crossdated fire-scarred trees (85%).
4.1 How have forest densities changed through time?
Using the crossdated trees from the 21 plots we reconstructed stand densities back through time (Figure 4.2). The common pattern is one of increasing forest densities, especially after ~1900. We urge some caution in interpreting very low stand densities during the earliest part of these reconstructions, as we expect to lose tree-ring material through time60. As trees die, the wood can be lost to decomposition and fire, and all that we are able to sample is what remained on the landscape when we visited the plots in 2016. We do have high confidence in the reconstructions through at least the middle part of the 1800s, and in some cases longer. This allows us to examine the differences in stand densities between now and during a time of frequent fire activity (Table 4.1). We found that all sites increased in forest densities, with the middle and low elevation sites [Tohatchi Lookout (TLK) and Piney Hill (PNH)] showing the most dramatic change. At PNH, densities more than doubled since 1870, and at TLK densities more than tripled. These changes are due to the influx of tree cohorts that established after frequent fire activity had ended. The lack of fire during the lifetimes of these trees substantially increased survivorship, resulting in forests of greater densities.
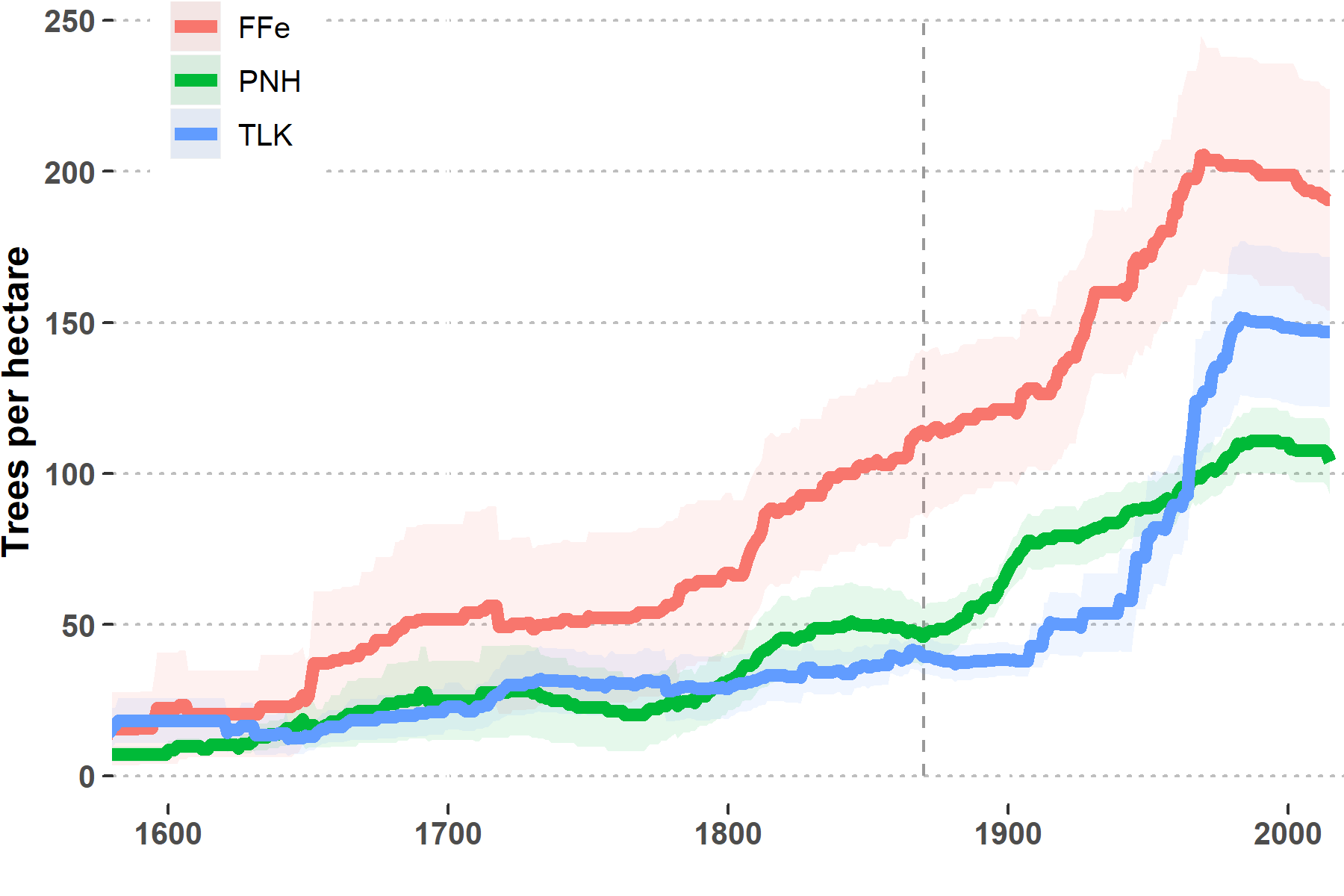
Figure 4.2: Reconstructed forest densities of the Navajo demography sites. The dotten line indicates 1870, the approximate end of frequent fire activity across Navajo forests. Shaded areas represent standard errors. (Note that 100 tph = ~40 tpa)
Site | 1870 | 2010 | % change |
---|---|---|---|
FFe | 113 | 193 | 70 |
PNH | 46 | 108 | 133 |
TLK | 40 | 148 | 273 |
4.2 How did drought and frequent fire activity influence regeneration?
At each of the demography sites, we found that tree cohorts of predominantly ponderosa pine established in the midst of ongoing and recurrent fire activity. We reconstructed historical fires for each site using the collection of fire-scarred trees, focusing on years with widespread fire activity (ie. years in which fire scarred at least 25% of sample trees). We compared the recruitment patterns to Palmer Drought Severity Index (PDSI), a commonly-used index of summer soil moisture. For the Navajo study area, we averaged four gridpoints of tree-ring based PDSI reconstructions from the North American Drought Atlas54. These methods follow 64 and have been successfully employed in other forests similar to Navajo65,70.
At the FFe demography site (Figure 4.3), major tree cohorts established in the mid-1600s, ~1800, and the late 1860s. There is near-continuous establishment of ponderosa pine and Douglas-fir at FFe during the 1800s, when fire activity significantly slowed down due to grazing by livestock and horses. The pulse of recruitment in 1800 at FFe was found mostly at a single plot and may represent mixed-severity effects of fire in 180169. Interestingly, aspen recruitment at FFe occurred in the absence of fire during the 20th century. Aspen is widely known to signal higher-severity fire activity because it resprouts prolifically after fire71,72.
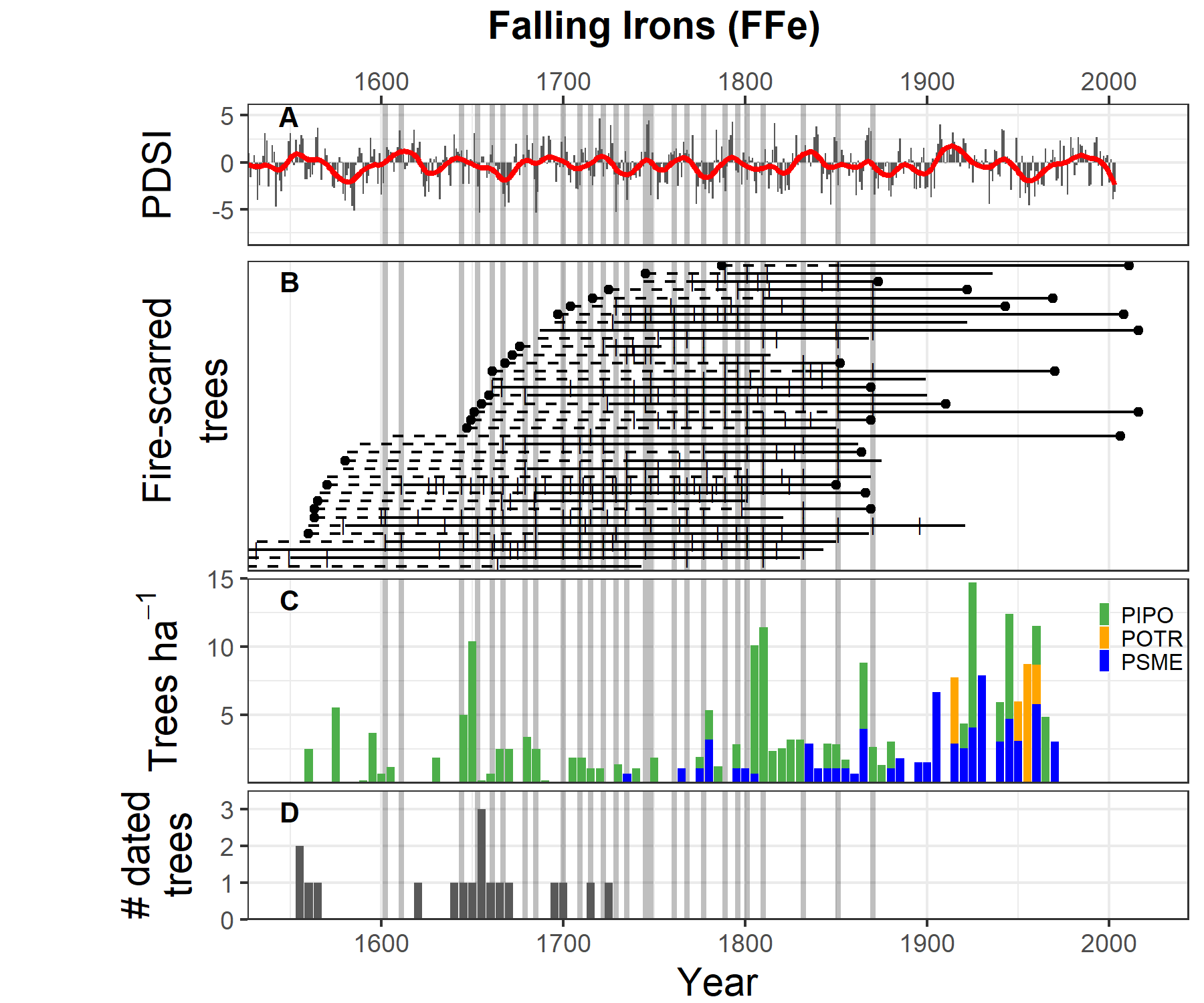
Figure 4.3: Comparison of climate, fire, and tree recruitment at Falling Irons. Panels include (A) tree-ring based PDSI, where positive values are wet and negative are dry; (B) fire-scarred trees, where each horizontal line represents a tree’s lifespan with the solid portion showing the time period of reliable fire recording, the vertical dashed are fire scars, and triangles are other injuries; (C) tree recruitment in 5-yr bins (10 tph = ~ 4 tpa); and (D) recruitment of trees from the site collected outside of plots for fire history or chronology purposes. In all panels, years of widespread fires (>25% trees scarred) are indicated with vertical grey bars.
At the PNH demography site (Figure 4.4), we found even more distinct cohorts than at FFe, but trees were able to nearly continuously recruit into the forest in the presence of frequent, widespread fires. Several tree cohorts established in the mid- to late-1600s under regionally wet conditions56. This is followed by a hiatus in recruitment through much of the 1700s when fire events were common. The largest and most prolonged recruitment pulse during the historical era at PNH occurred from 1770 to 1830. Although fires occurred during this period, they may have been of relatively low intensity to allow so many surviving trees. This late-18th to early-19th century has received much research because it appears to be an era of low variability in El Niño Southern Oscillation56. The period is known for reduced fire activity across many mountain ranges of the Southwest60, but fire activity did not change in Navajo forests44.
The last widespread fire at PNH occurred in 1879, consistent with other sites on the Defiance Plateau. Tree recruitment accelerated after this point, continuing through ~1910. Then another hiatus in recruitment ensued until ~1940 when a final prolonged pulse adds young trees to the stand through the 1980s (and possibly continuing, though we did not sample trees smaller than 10 cm DBH). These two late cohorts show the influences of a lack of fire to promote new trees into the forest (after 1879) and then the likely influence of grazing pressure during the 1910s. The 1910s is known for abundant ponderosa pine regeneration in the region61,62, and yet we found no trees recruiting at PNH in this decade. We suspect grazing pressures probably reduced the survivorship of seedlings through trampling, compaction of the seedbed, and some consumption because this era included relatively high levels of grazing50. Following the stock reduction program of the 1930s and 1940s, grazing pressures were dramatically reduced, allowing for another prolonged pulse of tree regeneration and recruitment, especially during relatively favorable climate of the 1940s and 1960s-1980s.
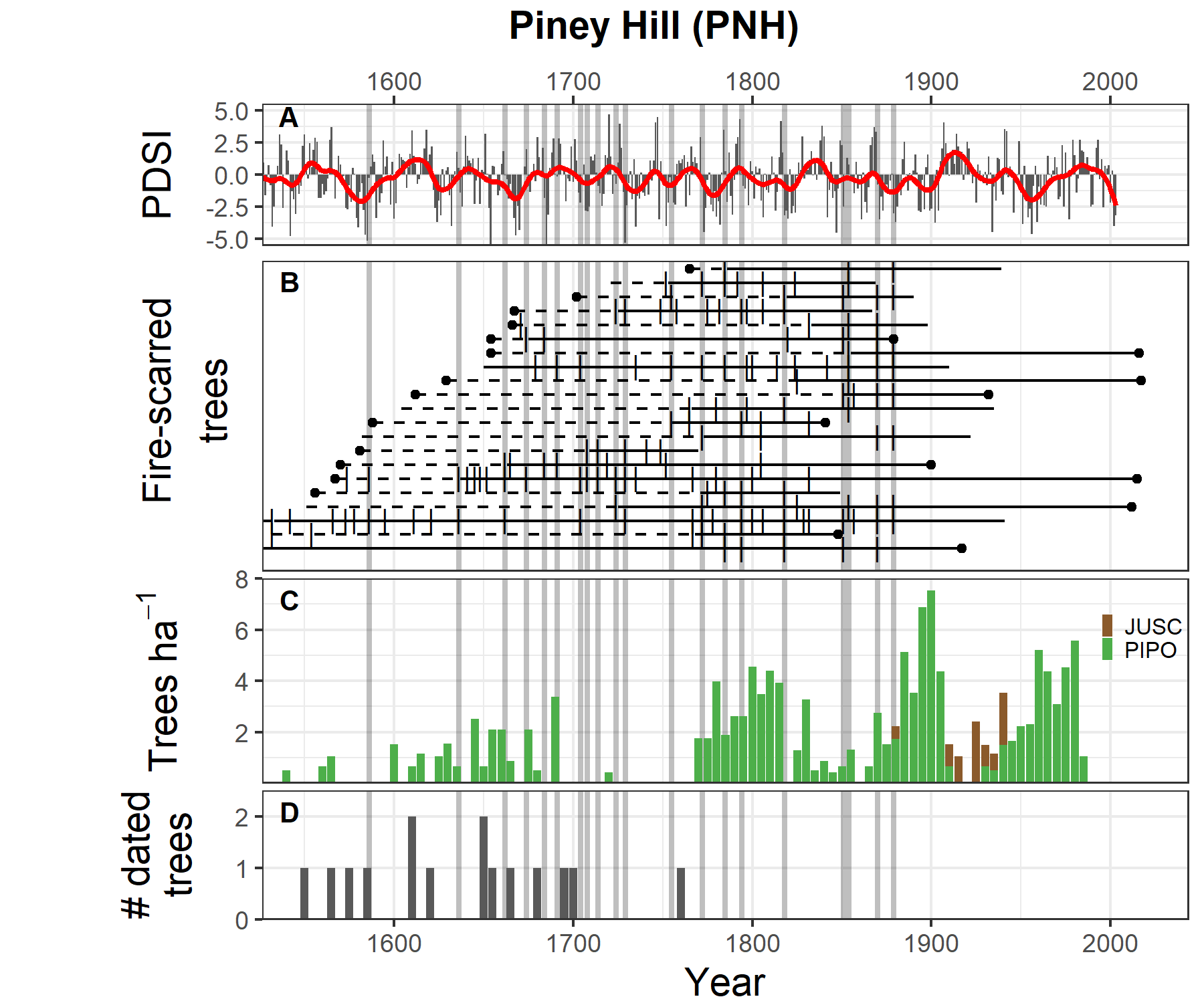
Figure 4.4: Comparison of climate, fire, and tree recruitment at Piney Hill. Panels are the same as above.
At the Tohatchi Lookout site (Figure 4.5), the major distinguishing feature is a large pulse of recruitment from the 1940s through the 1980s. One 5-year period in this pulse (1965-1970) had twice the average recruitment rate of the rest of the pulse period. It may relate to logging of the site, but that could not be confirmed. Like PNH, the 20th century recruitment pattern at TLK includes an early- to mid-century hiatus that precedes the stock reduction program. This adds further evidence to livestock effecting and/or removing tree regeneration, at least when livestock numbers are high. However, regeneration levels during the early-1900s were not far above the historical pattern. Historically, TLK had lower levels of cohort recruitment than at the other sites, but TLK shows fairly steady recruitment from the 1600s through the mid-1800s. A couple of small pulses of recruitment occurred in 1650 and 1715, but otherwise recruitment was low and gradual, occurring in the midst of highly frequent, widespread fire activity. There is a subtle rise in the density of new cohorts from 1845 to 1865 as fire activity began to slow down from the introduction and development of livestock grazing practices across the Chuska Mountains and including the TLK site. The last widespread fire is 1879, but increased recruitment is delayed until after 1900, presumably due to ongoing grazing.
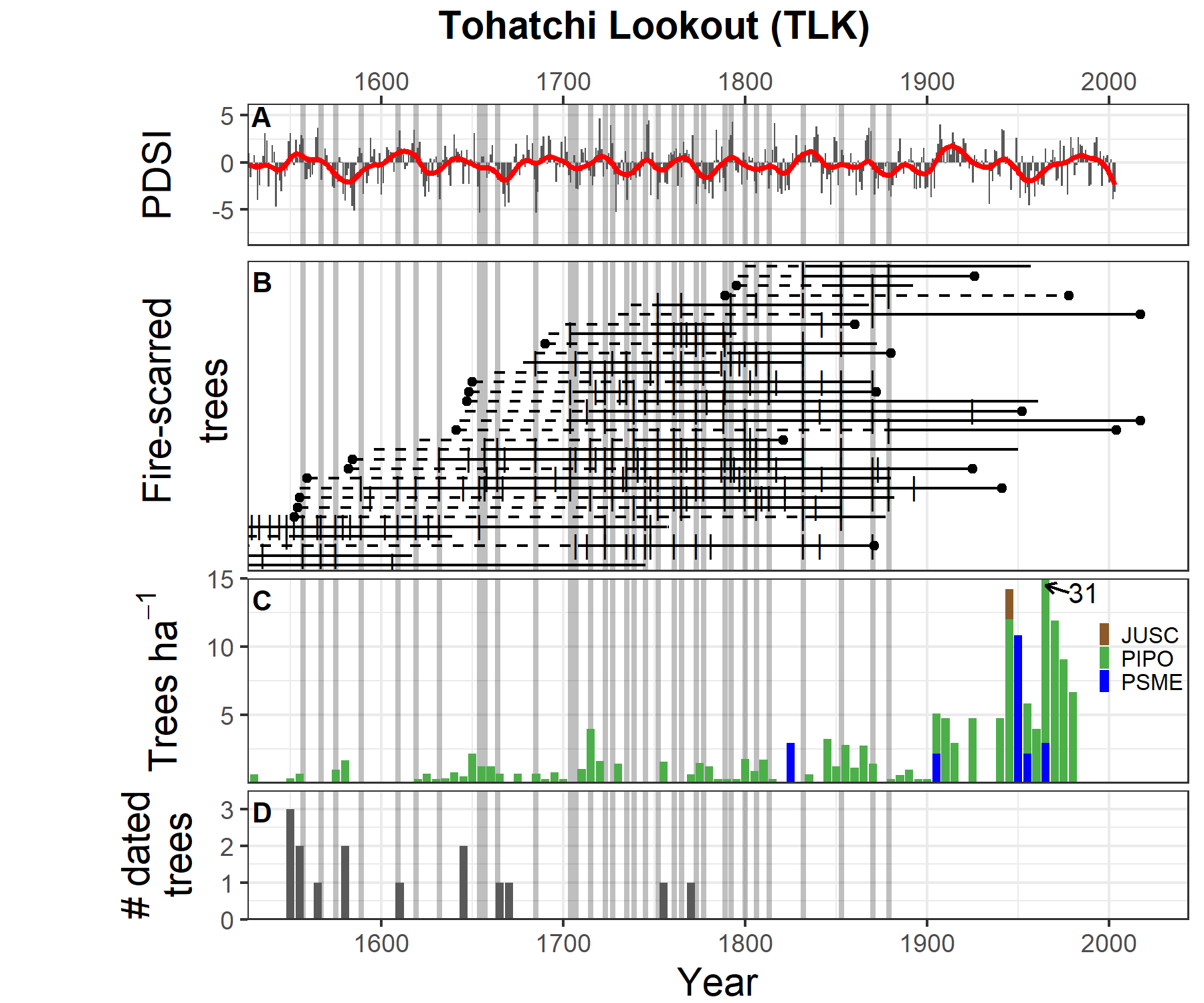
Figure 4.5: Comparison of climate, fire, and tree recruitment at Tohatchi Lookout. Panels are the same as above.
4.3 Conclusions
Ponderosa pine forests on the Navajo Nation sustained themselves in the presence of recurrent surface fire activity for hundreds of years. Despite the strong potential for fire to kill seedlings, many trees survived by directly resisting fire or being lucky enough to avoid it until adulthood. These findings demonstrate that surface fires today do not pose a significant threat to regeneration, at least at stand to landscape scales.
As the region grows warmer and drier with climate change, recurrent burning may help to offset some of the risk that droughts pose to regeneration. Recurrent fires and other forest treatments can build multi-aged stands that work to promote regeneration in the partial shade of the canopy. Indeed, overstory trees have a climatic buffering effect that insulates from extreme temperatures and aridity73,74, meaning that sub-canopy conditions can be conducive to regeneration even if the overall climate is not. Climatic buffering offered by multi-storied stands may explain why several major pulses of regeneration at the demography sites occurred during significant droughts, such as at TLK during the 1950s and 1960s.
Our results also indicate that livestock grazing was likely to have affected forest stand structures and recruitment dynamics, in addition to affecting fire activity44. Across the Southwest, forest densities in ponderosa pine stands dramatically increased as fire regimes collapsed and favorable climate persisted through the 1910s. At our Navajo sites, however, fire regimes ended by 1880 and we did not find subsequent increased stand densities until after 1940 at most plots (Figure 4.2). We attribute this lag in recruitment to suppression effects of livestock on seedlings, which could have included consumption, trampling, and/or soil compaction. Following the mid-20th century reduction in livestock, many widespread tree cohorts got established and are still growing today. These increased stand densities present a significant hazard to Navajo forests because younger, shorter trees increase the potential for high-severity fire by acting as ladder fuels carrying fires up into the crowns of large, otherwise fire-resistant trees.
References
12. Zeng, X., Broxton, P. & Dawson, N. Snowpack Change From 1982 to 2016 Over Conterminous United States. Geophysical Research Letters 2018GL079621 (2018). doi:10.1029/2018GL079621
59. Falk, D. Are Madrean ecosystems approaching tipping points? Anticipating interactions of landscape disturbance and climate change. in Merging science and management in a rapidly changing world: Biodiversity and management of the madrean archipelago iii; 2012 may 1-5; tucson, az. proceedings. rmrs-p-67. fort collins, co (eds. Gottfried, G., Ffolliott, P., Gebow, B., Eskew, L. & Collins, L.) 40–47 (U.S. Department of Agriculture, Forest Service, Rocky Mountain Research Station., 2013).
60. Swetnam, T. W. & Brown, P. M. Climatic inferences from dendroecological reconstructions. in Dendroclimatology: Progress and prospectives. developments in paleoenvironmental research (eds. Hughes, M. K., Diaz, H. F. & Swetnam, T. W.) 263–295 (Springer Verlag, 2011). doi:10.1007/978-1-4020-5725-0
61. Pearson, G. Natural reproduction of western yellow pine in the Southwest. (1923).
62. Savage, M., Brown, P. & Feddema, J. The role of climate in a pine forest regeneration pulse in the southwestern United States. Ecoscience 3, 310–318 (1996).
63. White, A. Presettlement regeneration patterns in a southwestern ponderosa pine stand. Ecology 66, 589–594 (1985).
64. Brown, P. M. & Wu, R. Climate and disturbance forcing of episodic tree recruitment in a southwestern ponderosa pine landscape. Ecology 86, 3030–3038 (2005).
65. Meunier, J., Brown, P. & Romme, W. Tree recruitment in relation to climate and fire in northern Mexico. Ecology 95, 197–209 (2014).
44. Guiterman, C. H. Climate and human drivers of forest vulnerability in the US Southwest: Perspectives from dendroecology. 185 (University of Arizona, 2016).
66. Speer, J. H. Fundamentals of tree-ring research. 333 (The University of Arizona Press, 2010).
67. Applequist, M. A simple pith locator for use with off-center increment cores. Journal of Forestry 51, 141 (1958).
68. Margolis, E. Q. Fire regime shift linked to increased tree density in a pinon-juniper landscape. International Journal of Wildland Fire 23, 234–245 (2014).
69. Margolis, E. Q. & Malevich, S. B. Historical dominance of low-severity fire in dry and wet mixed-conifer forest habitats of the endangered terrestrial Jemez Mountains salamander (Plethodon neomexicanus). Forest Ecology and Management 375, 12–26 (2016).
41. Baisan, C. & Swetnam, T. Fire history on a desert mountain range: Rincon Mountain Wilderness, Arizona, USA. Canadian Journal of Forest Research 20, 1559–1569 (1990).
54. Cook, E. R. & Krusic, P. J. The North American Drought Atlas. (2004).
70. Brown, P. M. & Cook, B. Early settlement forest structure in Black Hills ponderosa pine forests. Forest Ecology and Management 223, 284–290 (2006).
71. Margolis, E. Q., Swetnam, T. W. & Allen, C. D. A stand-replacing fire history in upper montane forests of the southern Rocky Mountains. Canadian Journal of Forest Research 37, 2227–2241 (2007).
72. Tepley, A. J. & Veblen, T. T. Spatiotemporal fire dynamics in mixed-conifer and aspen forests in the San Juan Mountains of southwestern Colorado, USA. Ecological Monographs 85, 583–603 (2015).
56. Swetnam, T. W. & Betancourt, J. L. Mesoscale disturbance and ecological response to decadal climatic variability in the American Southwest. Journal of Climate 11, 3128–3147 (1998).
50. Weisiger, M. Dreaming of sheep in Navajo country. (University of Washington Press, 2009).
73. Frey, S. J. et al. Spatial models reveal the microclimatic buffering capacity of old-growth forests. Science Advances 2, (2016).
74. Davis, K. T., Dobrowski, S. Z., Holden, Z. A., Higuera, P. E. & Abatzoglou, J. T. Microclimatic buffering in forests of the future: the role of local water balance. Ecography (2018). doi:10.1111/ecog.03836