Section 5 Tree growth response to climate
Ongoing drought conditions in the Southwest raise concerns about declining tree growth and forest productivity. Growth declines attributed to warm, dry conditions have already been documented across the Southwest and parts of the western US, and are predicted to worsen over the next 20-30 years with climate change15,27,31,75,76. Declining tree growth is of concern because it would lead to substantial losses of forest productivity, carbon sequestration, and ecosystem services20,77,78.
In the Southwest, tree growth is primarily limited by soil moisture79. Temperature influences the availability of soil moisture by drawing water from soil and plants and into the atmosphere. The atmospheric demand for water rises exponentially as temperature increases9,15. This exasperates drought, even turning normal conditions into a drought. From a tree perspective, a warming climate means that average years in the future will be like the worst drought years of the past15.
How future drought conditions manifest across the region depends largely on the characteristics of individual landscapes. Variations in topography, soil type, soil depth, and elevation impart differential responses of trees to climate80. Trees with low climate sensitivity (those that grow well during dry years) tend to resist disturbances more effectively than climatically stressed individuals30,81. Therefore, understanding where on the landscape trees are more versus less sensitive to climate is key to understanding landscape-scale vulnerability to climate change.
Here, we examine patterns of tree growth across the Navajo forest. We quantify climate sensitivity and map its spatial distribution, and then evaluate the role of climate in changing patterns of tree growth. Finally, we assess whether growth declines have occurred in Navajo forests due to the current, ongoing drought.
5.2 What explains the landscape variability in climate sensitivity?
Among the many topographic and climate variables that might help to explain the landscape variation in climate sensitivity, we found that elevation did the best job (Figure 5.4). Elevation captures changes in precipitation (higher is wetter), temperature (higher is cooler), and VPD (higher is lower), and is positively correlated with multiple metrics of forest structure like density and basal area across the CFI plots. Changes in elevation explain nearly half of the variation in climate sensitivity for ponderosa pine, our best-sampled species. If we could add plots for Douglas-fir and piñon, we might find similar patterns. But in general, as elevation increases, sensitivity to climate decreases for both ponderosa pine and Douglas-fir. For piñon, there’s no elevation trend, but as we noted, our sample of piñon is only the upper-elevation slice of its overall distribution. It is possible that lower-elevation piñon may be more sensitive to climate, resulting in similar trends as for the other species.
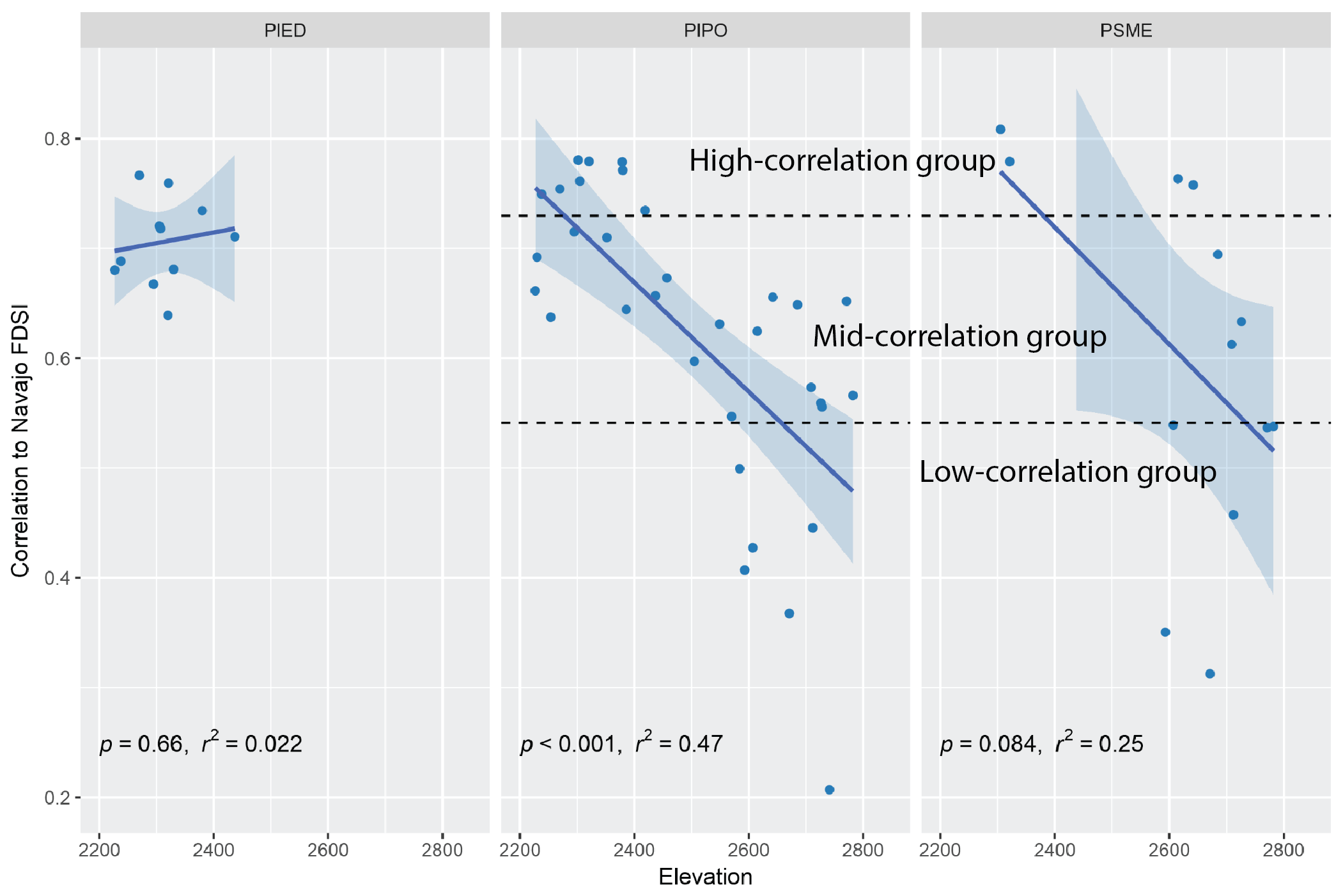
Figure 5.4: Climate sensitivity as a function of elevation for CFI plots. Each panel presents a difference species: PIED piñon; PIPO ponderosa pine; PSME Douglas-fir. The PIPO and PSME were divided into three categories based on the strength of correlation.
To better understand differences among CFI plots with regard to drought impacts on tree growth, we grouped the plots based on their sensitivity to climate. We created four groups, as seen in Figure 5.4: piñon (PIED), high-correlation ponderosa pine and Douglas-fir (HighCor), mid-correlation ponderosa pine and Douglas-fir (MidCor), and low-correlation ponderosa pine and Douglas-fir (LowCor). Although these groups are based on climate sensitivity, they also reflect general patterns in the elevational distribution of plots. Higher-elevation, lower-correlation and lower-sensitivity plots are in the LowCor group, while lower elevation, more climate-sensitive plots are in the HighCor group. The MidCor group spans the elevation range, and is likely influenced by other topographic factors such as solar radiation and soil quality/depth.
The growth patterns within and among these groups are quite similar, and it is clear from the tree growth patterns presented in Figure 5.5 that the PIED and HighCor groups have the greatest variability in tree growth, reflecting their high climate sensitivity. One pattern sticks out here that deserves further attention: since roughly 1985, the growth variability of the LowCor, higher-elevation trees has changed markedly. It has greater variability across all plots and appears to also have greater synchrony between plots. These changes could indicate change climate sensitivity that would have to be related to recent change sin the climate.
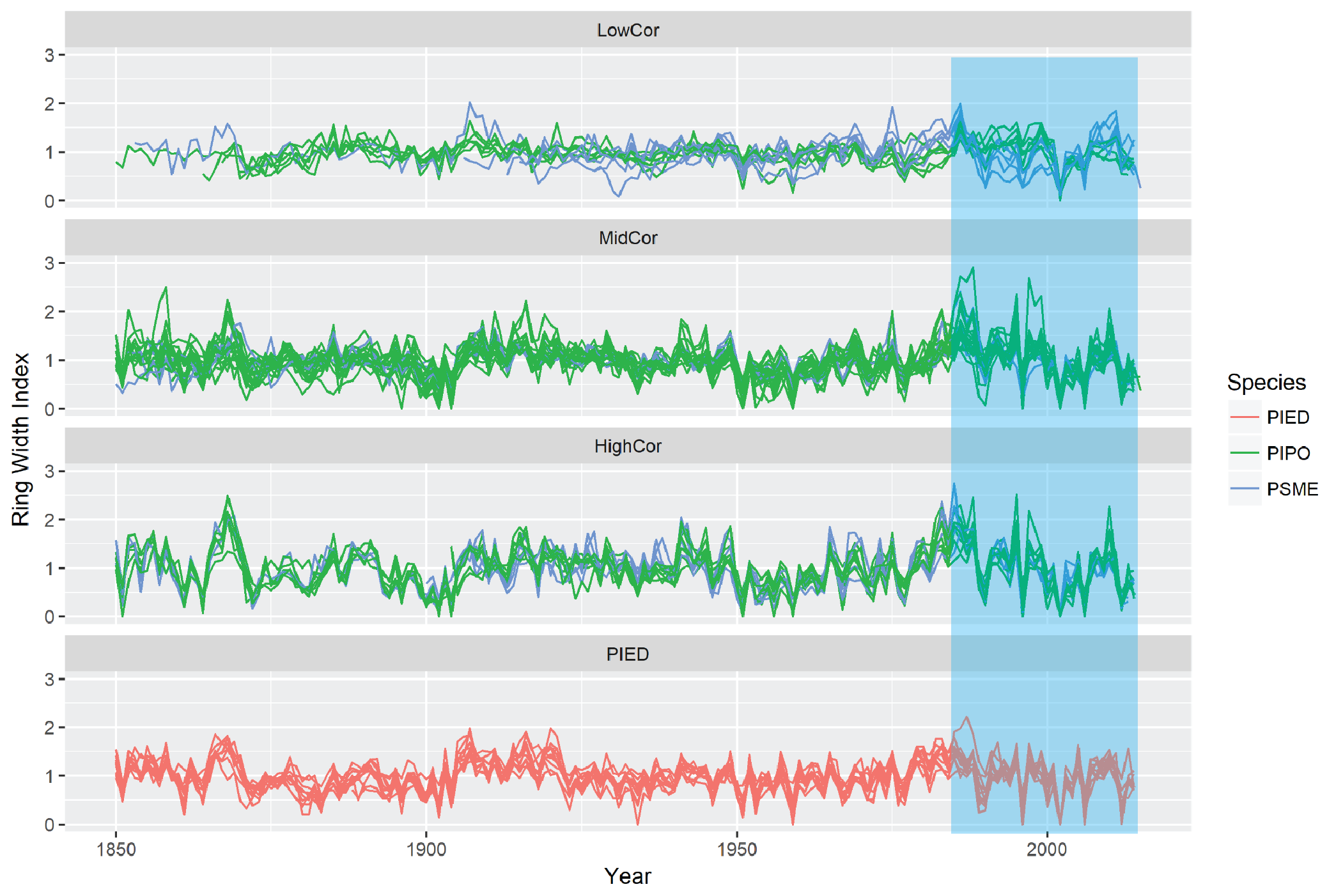
Figure 5.5: Growth patterns of CFI plots, divided by species and strength of correlation. The blue shaded area represents the period since ~1985 in which growth character has changed with climate change.
We tested the degree to which our observation of changing growth pattern at the upper-elevation LowCor plots is real and biologically meaningful. This was done in two ways: First, we assessed a metric called Express Population Signal, or EPS, that quantifies how well the growth patterns between different plots are synchronized (Figure 5.6). Greater synchrony in growth indicates higher overall response to climate, as it is climate that regulates synchrony in tree growth. And indeed, the last 30-year growth period (1986-2016) has an EPS value in the LowCor group that is higher than anything since 1900, and is within the long-term range of the other groups. We found upward trends in EPS to be significant for both the LowCor and MidCor groups, based on regression analysis.
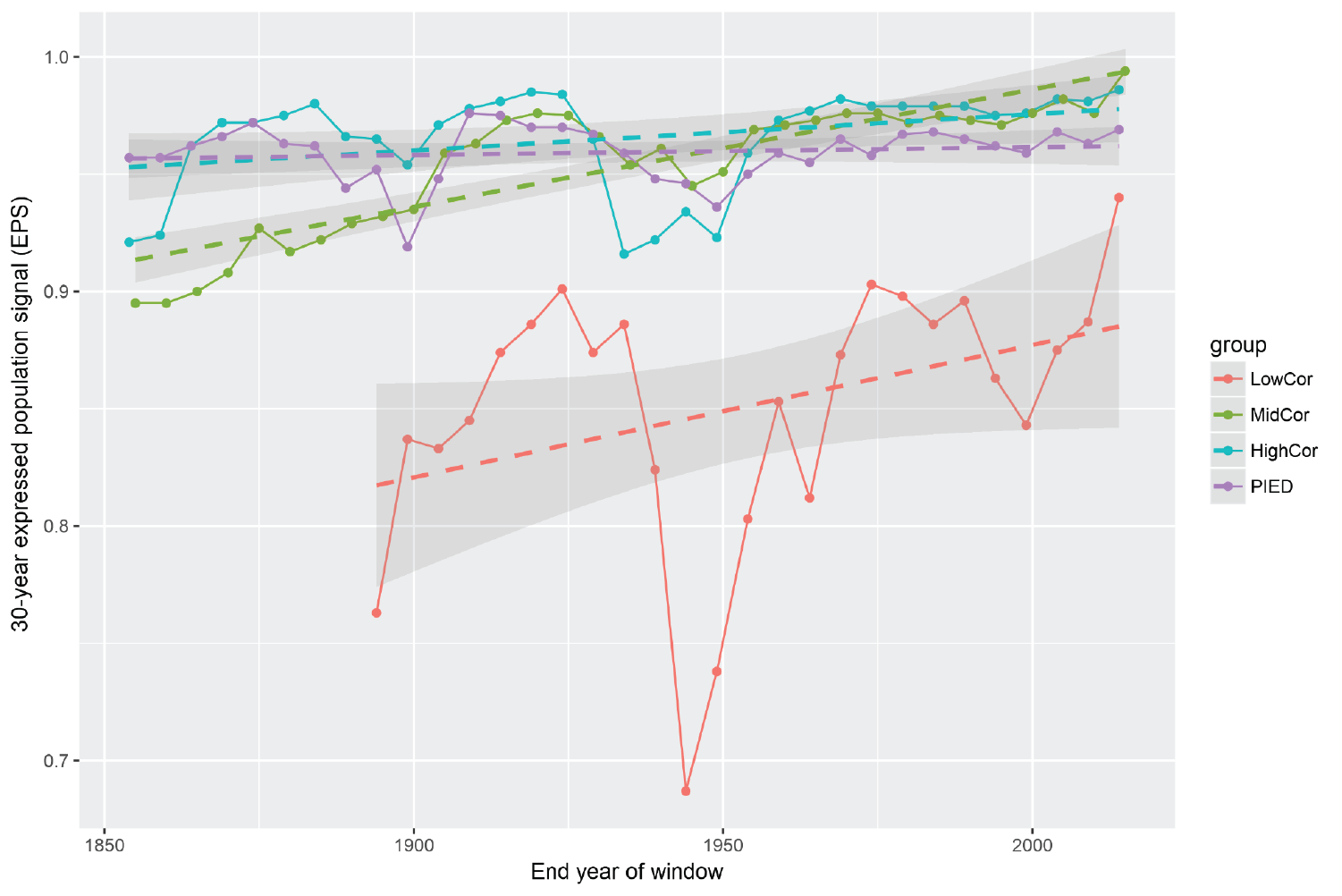
Figure 5.6: Comparison of growth synchrony among different groups of CFI plots. Solid lines show the running-window EPS values. Dotted lines represent linear regression of the patterns with shaded areas showing the 95% confidence interval around the regression.
In the second test of changing growth patterns, we found that the average growth variability (standard deviation) rapidly increased across the LowCor plots since about 1950 (Figure 5.7). This metric of growth variability corresponds to the strength of climate in driving tree growth, wherein higher standard deviations of tree growth are more climatically sensitive31. Growth variability across all CFI plots fluctuated over time, but no other group showed such a dramatic increase in standard deviation as that of the LowCor group in recent decades. Furthermore, the variability in growth at LowCor plots remained well below that of the other groups throughout the 1900s, but is now within the range of the MidCor and PIED groups. This change indicates that conditions for tree growth at the higher elevations of the Chuska Mountains are now somewhat equivalent to historical levels of the lower elevations.
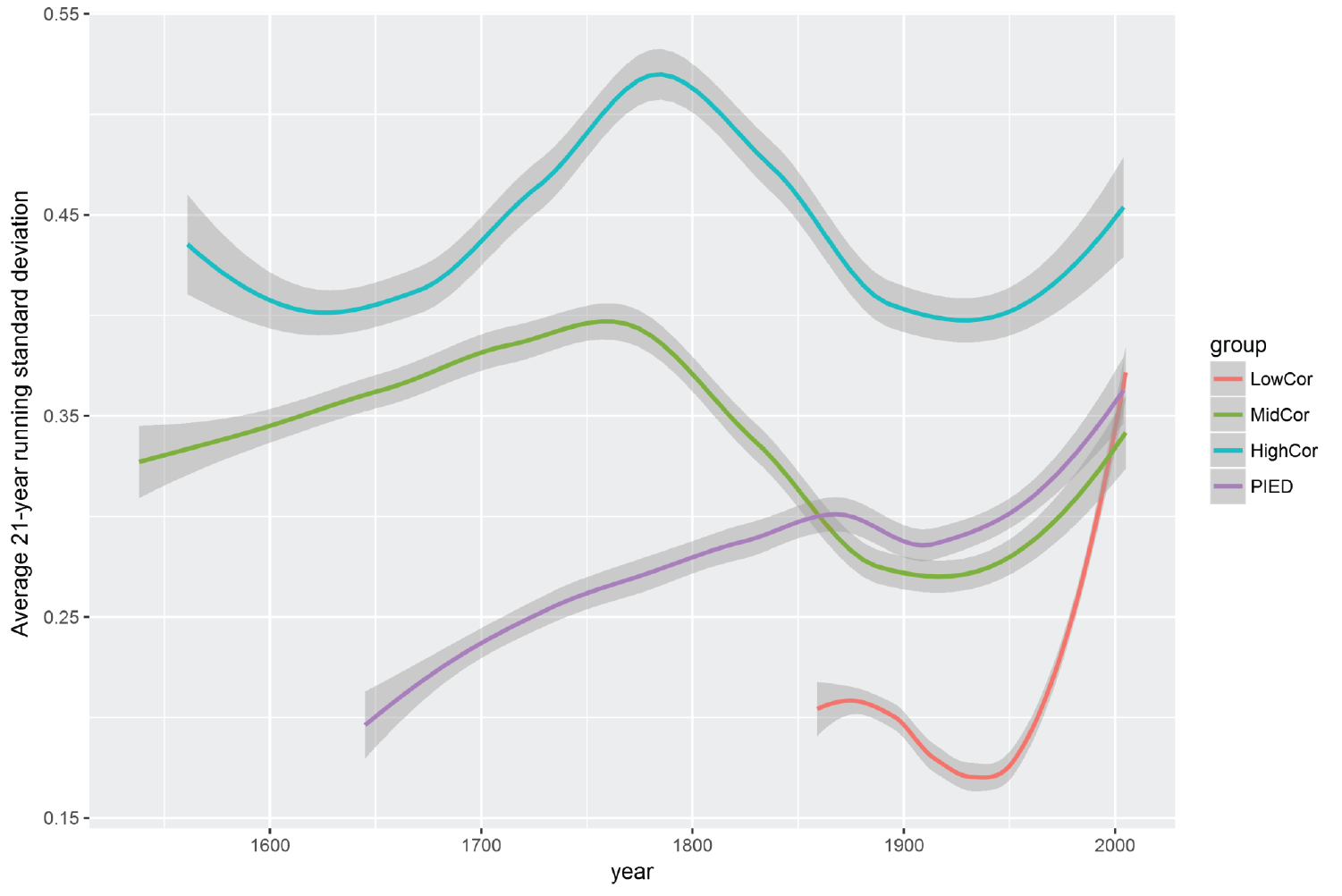
Figure 5.7: Smoothed trends in forest growth variability among the CFI plot groups. Shaded areas show the 95% confidence intervals.
5.4 Conclusions
Across the Navajo forest, we found substantial variability in the response of tree growth to climate. Across all CFI plots, climate plays a role in limiting soil moisture for growth, with lower-elevation areas such as the Defiance Plateau most highly regulated by climate. In general, upper-elevation sites composted of dry mixed conifer forests (mixed ponderosa pine and Douglas-fir) have the lowest sensitivity to climate. This is due to higher precipitation and lower temperatures on average that maintain sufficient soil moisture throughout the growing season.
Although lower elevation areas are highly sensitive to climate, we did not find strong evidence for declining growth over the warmer last few decades. Growth rates (BAI) were lower in the 2000s than they were in the 1980s, but we attribute this change to differences between a wet decade and dry one, with growth rates since the mid-1990s returning to close to the 20th century average.
An alarming trend that we found is the increasing level of climate sensitivity at the upper-elevation plots. This trend was quantified in two ways - growth synchrony and growth variability, and is notable in both standardized ring width indices and BAI. The changes indicate that upper-elevation trees, which were once buffered from drought episodes such as the 1950s, have recently exhibited the effects of drought due to rising temperatures since the mid-1980s. Rising temperatures appear to have begun to limit soil moisture during the growing season, making the trees more responsive to climate variability and winter precipitation than they probably ever were before. Although overall growth rates have not declined with these shifting climate sensitivity, it signals the potential for changes in the future. It also shows that climate change has progressed in a steady upward march in the Chuska Mountains, turning tree growth patterns at the upper-elevations into something akin to mid and lower elevations.
A component to alarm raised by these findings is the steady loss of landscape diversity in reponse to drought and disturbance. Climate change is making tree growth more homogeneous at the landscape-scale. Previously, when a drought or widespread disturbance (fire or insect outbreak) occurred, much of the area of the Navajo forest would be buffered from its effects by the resistance garnered from plentiful resources, especially water. The LowCor, upper-elevation plots represent ~20% of the Navajo forest. But now, hotter droughts have synchronized growth response closer to a level where landscape diversity may be lost. Disturbances may be able to affect much greater areas. Our findings thus indicate that climate change is reducing landscape variability in the resistance of trees to drought.
References
15. Williams, A. P. et al. Temperature as a potent driver of regional forest drought stress and tree mortality. Nature Climate Change 3, 292–297 (2013).
27. Williams, A. P. et al. Forest responses to increasing aridity and warmth in the southwestern United States. Proceedings of the National Academy of Sciences of the United States of America 107, 21289–94 (2010).
31. Klesse, S. et al. Sampling bias overestimates climate change impacts on forest growth in the southwestern United States. Nature Communications 9, 5336 (2018).
75. Restaino, C. M., Peterson, D. L. & Littell, J. Increased water deficit decreases Douglas fir growth throughout western US forests. Proceedings of the National Academy of Sciences 113, 9557–9562 (2016).
76. Charney, N. D. et al. Observed forest sensitivity to climate implies large changes in 21st century North American forest growth. Ecology Letters 19, 1119–1128 (2016).
20. Allen, C. D., Breshears, D. D. & McDowell, N. G. On underestimation of global vulnerability to tree mortality and forest die-off from hotter drought in the Anthropocene. Ecosphere 6, art129 (2015).
77. Ogle, K., Whitham, T. G. & Cobb, N. S. Tree-ring variation in pinyon predicts likelihood of death following severe drought. Ecology 81, 3237–3243 (2000).
78. Anderegg, W. R. L., Kane, J. M. & Anderegg, L. D. L. Consequences of widespread tree mortality triggered by drought and temperature stress. Nature Climate Change (2012). doi:10.1038/nclimate1635
79. St. George, S. An overview of tree-ring width records across the Northern Hemisphere. Quaternary Science Reviews 95, 132–150 (2014).
9. Breshears, D. D. et al. The critical amplifying role of increasing atmospheric moisture demand on tree mortality and associated regional die-off. Frontiers in plant science 4, 266 (2013).
80. Fritts, H. C., Smith, D. G., Cardis, J. W. & Budelsky, C. A. Tree-ring characteristics along a vegetation gradient in northern Arizona. Ecology 46, 393–401 (1965).
30. van Mantgem, P. J., Falk, D. A., Williams, E. C., Das, A. J. & Stephenson, N. L. Pre-fire drought and competition mediate post-fire conifer mortality in western U.S. National Parks. Ecological Applications 28, 1730–1739 (2018).
81. Macalady, A. K. & Bugmann, H. Growth-mortality relationships in pinon pine (Pinus edulis) during severe droughts of the past century: Shifting processes in space and time. PLoS ONE 9, (2014).